Growth And Reproduction Study Guide
- Reproduction And Growth In Plants
- Cell Growth And Reproduction Study Guide Answers
- Cell Growth And Reproduction Study Guide
The cell cycle is generally divided into two phases: interphase and mitosis. During interphase, the cell spends most of its time performing the functions that make it unique. Mitosis is the phase of the cell cycle during which the cell divides into two daughter cells. Interphase The interphase stage of the cell cycle includes three distinctive parts: the G 1 phase, the S phase, and the G 2 phase.
The G 1 phase follows mitosis and is the period in which the cell is synthesizing its structural proteins and enzymes to perform its functions. For example, a pancreas cell in the G 1 phase will produce and secrete insulin, a muscle cell will undergo the contractions that permit movement, and a salivary gland cell will secrete salivary enzymes to assist digestion. During the G 1 phase, each chromosome consists of a single molecule of DNA and its associated histone protein. In normal human cells, there are 46 chromosomes per cell (except in sex cells with 23 chromosomes and red blood cells with no nucleus and, hence, no chromosomes). During the S phase of the cell cycle, the DNA within the nucleus replicates.
During this process, each chromosome is faithfully copied, so by the end of the S phase, two DNA molecules exist for each one formerly present in the G 1 phase. Human cells contain 92 chromosomes per cell in the S phase. In the G 2 phase, the cell prepares for mitosis. Proteins organize themselves to form a series of fibers called the spindle, which is involved in chromosome movement during mitosis. The spindle is constructed from amino acids for each mitosis, and then taken apart at the conclusion of the process. Spindle fibers are composed of microtubules. Mitosis The term mitosis is derived from the Latin stem mito, meaning “threads.” When mitosis was first described a century ago, scientists had seen “threads” within cells, so they gave the name “mitosis” to the process of “thread movement.” During mitosis, the nuclear material becomes visible as threadlike chromosomes.
The chromosomes organize in the center of the cell, and then they separate, and 46 chromosomes move into each new cell that forms. Mitosis is a continuous process, but for convenience in denoting which portion of the process is taking place, scientists divide mitosis into a series of phases: prophase, metaphase, anaphase, and telophase (see Figure 7-1): Figure 7-1 The process of mitosis, in which the chromosomes of a cell duplicate and pass into two daughter cells. ■ Prophase: Mitosis begins with the condensing of the chromatin to form chromosomes in the phase called prophase. Two copies of each chromosome exist; each one is a chromatid. Two chromatids are joined to one another at a region called the centromere.
As prophase unfolds, the chromatids become visible in pairs (called sister chromatids), the spindle fibers form, the nucleoli disappear, and the nuclear envelope dissolves. In animal cells during prophase, microscopic bodies called centrioles begin to migrate to opposite sides of the cell. When the centrioles reach the poles of the cell, they produce and are then surrounded by a series of radiating microtubules called an aster. Centrioles and asters are not present in most plant or fungal cells. As prophase continues, the chromatids attach to spindle fibers that extend out from opposite poles of the cell. The spindle fibers attach at the region of the centromere at a structure called the kinetochore, an area of protein in the centromere region. Eventually, all pairs of chromatids reach the center of the cell, a region called the equatorial plate.
■ Metaphase: Metaphase is the stage of mitosis in which the pairs of chromatids line up on the equatorial plate. This region is also called the metaphase plate. In a human cell, 92 chromosomes in 46 pairs align at the equatorial plate. Each pair is connected at the centromere, where the spindle fiber is attached (more specifically at the kinetochore). ■ Anaphase: At the beginning of anaphase, the sister chromatids move apart from one another. The chromatids are called chromosomes after the separation.
Each chromosome is attached to a spindle fiber, and the members of each chromosome pair are drawn to opposite poles of the cell by the spindle fibers. During anaphase, the chromosomes can be seen moving. They take on a rough V shape because of their midregion attachment to the spindle fibers. The movement toward the poles is accomplished by several mechanisms, such as an elongation of the spindle fibers, which results in pushing the poles apart. The result of anaphase is an equal separation and distribution of the chromosomes. In human cells, a total of 46 chromosomes move to each pole as the process of mitosis continues. ■ Telophase: In telophase, the chromosomes finally arrive at the opposite poles of the cell.
The distinct chromosomes begin to fade from sight as masses of chromatin are formed again. The events of telophase are essentially the reverse of those in prophase. The spindle is dismantled and its amino acids are recycled, the nucleoli reappear, and the nuclear envelope is reformed. ■ Cytokinesis: Cytokinesis is the process in which the cytoplasm divides and two separate cells form. Note that cytokinesis is separate from the four stages of mitosis. In animal cells, cytokinesis begins with the formation of a cleavage furrow in the center of the cell.
With the formation of the furrow, the cell membrane begins to pinch into the cytoplasm, and the formation of two cells begins. This process is often referred to as cell cleavage.
Microfilaments contract during cleavage and assist the division of the cell into two daughter cells. In plant cells, cytokinesis occurs by a different process because a rigid cell wall is involved. Cleavage does not take place in plant cells. Rather, a new cell wall is assembled at the center of the cell, beginning with vesicles formed from the Golgi apparatus (see Chapter 3). As the vesicles join, they form a double membrane called the cell plate.
The cell plate forms in the middle of the cytoplasm and grows outward to fuse with the cell membrane. The cell plate separates the two daughter cells.
As cell wall material is laid down, the two cells move apart from one another to yield two new daughter cells. Mitosis serves several functions in living cells. In many simple organisms, it is the method for asexual reproduction (for example, in the cells of a fungus). In multicellular organisms, mitosis allows the entire organism to grow by forming new cells and replacing older cells.
In certain species, mitosis is used to heal wounds or regenerate body parts. It is the universal process for cell division in eukaryotic cells.
TED SALMON Each of us began as a single cell. This cell couldn't move, think, see, or do things like laugh and talk. But the one thing it could do, and do very well, was divide—and divide it did. The lone cell became two, and then four, then eight and so on, in time becoming the amazing person that is you. Think of how far you've come. You can laugh at a joke, stand on your head, read a book, eat an ice cream cone, hear a symphony, and do countless other things.
In this chapter, we will discuss how cells divide, a topic that has fascinated scientists since they first observed it through a microscope more than 100 years ago. Scientists can actually watch cells divide under the microscope, and they have been able to figure out the rules of division by carefully observing the process, much as someone could gradually learn the rules of a game like football or chess by watching it played repeatedly. But you don't need your own microscope to see cells dividing.
By hooking up cameras to their microscopes, scientists have produced stunning images of the process, some of which we've reproduced here. TORSTEN WITTMAN There are two kinds of cell division: and. Mitosis is essentially a duplication process: It produces two genetically identical 'daughter' cells from a single 'parent' cell. You grew from a single embryonic cell to the person you are now through mitosis.
Reproduction And Growth In Plants
Even after you are grown, mitosis replaces cells lost through everyday wear and tear. The constant replenishment of your skin cells, for example, occurs through mitosis. Mitosis takes place in cells in all parts of your body, keeping your tissues and organs in good working order. Meiosis, on the other hand, is quite different. It shuffles the genetic deck, generating daughter cells that are distinct from one another and from the original parent cell. Although virtually all of your cells can undergo mitosis, only a few special cells are capable of meiosis: those that will become eggs in females and sperm in males.
So, basically, mitosis is for growth and maintenance, while meiosis is for sexual reproduction. The Cycling Cell. Look here if you want to see a cell cycle.
Cell Growth And Reproduction Study Guide Answers
Before focusing on mitosis, let's take a step back and look at the big picture. The illustration shows the cell cycle of a eukaryotic plant or animal cell. This cycle begins when the cell is produced by mitosis and runs until the cell undergoes its own mitosis and splits in two. The cycle is divided into distinct phases: G 1 (gap 1) S (synthesis), G 2 (gap 2), and M (mitosis). As you can see, mitosis only occupies a fraction of the cycle.
The rest of the time-phases G 1 through G 2—is known as. Scientists used to think of interphase as a resting phase during which not much happened, but they now know that this is far from the truth.
It is during interphase that chromosomes—the genetic material—are copied, and cells typically double in size. While this is happening, cells continue to do their jobs: Your heart muscle cells contract and pump blood, your intestinal cells absorb the food you eat, your thyroid gland cells churn out hormones, and so on. In contrast, most of these activities cease during mitosis while the cell focuses on dividing. But as you have probably figured out, not all cells in an organ undergo mitosis at the same time.
While one cell divides, its neighbors work to keep your body functioning. At first glance, the orderly progression of the cell through the phases of the cell cycle may seem perfectly straightforward. When building a house, the walls aren't erected until after the foundation has been laid. Likewise, in the cell, mitosis doesn't begin until after the genetic material has been copied. Otherwise, the daughter cells would end up with less than a complete set of chromosomes and would probably die. So in the cell cycle, just as in housebuilding, certain steps need to precede others in an orderly fashion for the process to work.
How does the cell 'know' when a step has been completed and it's time to move on to the next? The answer is that the cell has several molecular 'inspectors' stationed at intervals—called —throughout the cell cycle. These cellular inspectors function much like building inspectors do: If a step has been completed to their satisfaction, they give the OK to move forward. If not, they halt progress until the cellular construction workers finish the task. There are three major checkpoints in the cell cycle: one between G 1 and S phase, one between G 2 and mitosis, and one during mitosis itself. The concept of checkpoints in the cell cycle was first introduced by Ted Weinert of the University of Arizona in Tucson, and Leland Hartwell of the Fred Hutchinson Cancer Research Center in Seattle, Washington.
In experiments with yeast cells, Weinert and Hartwell showed that a protein called Rad9 is part of a cell cycle checkpoint. Normal cells will stop and repair any damage to their DNA before embarking upon mitosis. Cells that lack Rad9, however, ignore the damage and proceed through mitosis, with catastrophic consequences—having inherited damaged DNA, the daughter cells invariably die.
Since these discoveries were made, other checkpoint genes have been identified in many kinds of cells, including human cells. Hartwell has identified more than 100 genes that help control the cell cycle, and in recognition of the importance of these discoveries, he shared the Nobel Prize in physiology or medicine in 2001. Phases of Mitosis. Interphase: Chromosomes duplicate, and the copies remain attached to each other. Prophase: In the nucleus, chromosomes condense and become visible. In the cytoplasm, the spindle forms. Prometaphase: The nuclear membrane breaks apart, and the spindle starts to interact with the chromosomes.
Metaphase: The copied chromosomes align in the middle of the spindle. Anaphase: Chromosomes separate into two genetically identical groups and move to opposite ends of the spindle. Telophase: Nuclear membranes form around each of the two sets of chromosomes, the chromosomes begin to spread out, and the spindle begins to break down. Cytokinesis: The cell splits into two daughter cells, each with the same number of chromosomes as the parent. In humans, such cells have two copies of 23 chromosomes and are called. Mitosis: Let's Split! Mitosis is the most dramatic event in a cell's life.
Cellular structures that have always been there suddenly disintegrate, new structures are constructed, and it all culminates in the cell splitting in half. Imagine quietly going about your business one day, when you suddenly feel the bones of your skeleton rearranging themselves. Then, you find yourself being pinched apart from your midline, and before you know it, someone who looks just like you is sitting beside you.
That's akin to what happens to a cell during mitosis. Mitosis is divided into six phases:, and. The first five phases do the job of splitting the nucleus and its duplicated genetic information in two, while in the final step, the entire cell is split into two identical daughter cells. Your body carefully controls which cells divide and when they do so by using molecular stop and go signals.
For example, injured cells at the site of a wound send go signals to the surrounding skin cells, which respond by growing and dividing and eventually sealing over the wound. Conversely, stop signals are generated when a cell finds itself in a nutrient-poor environment. Sometimes, however, go signals are produced when they shouldn't be, or stop signals aren't sent or heeded. Both scenarios can result in uncontrolled cell division and cancer. Mitosis then becomes a weapon turned against the body, spurring the growth of invasive tumors. Fortunately, it takes more than one mistaken stop or go signal for a cell to become cancerous.
Because our bodies are typically quite good at protecting their essential systems, it usually requires a one-two punch for healthy cells to turn malignant. The punches come in the form of errors, or, in DNA that damage a gene and result in the production of a faulty protein. Sunlight, X rays and other radiation, toxins such as those found in cigarette smoke and air pollution, and some viruses can cause such mutations. People also can inherit mutations from their parents, which explains why some families have higher rates of certain cancers: The first punch is delivered at conception.
Subsequent mutations can then push a cell down the path toward becoming cancerous. Ironically, mitosis itself can cause mutations too, because each time a cell's DNA is copied, errors are made. (Fortunately, almost all of these errors are corrected by our extremely efficient DNA repair systems.) So there's an inherent tradeoff in mitosis: It allows us to grow to maturity and keeps us healthy, but it's also the source of potentially damaging DNA mutations. We'll come back to the link between cell division and DNA damage when we talk about aging in the next chapter. Meiosis: Sex, Heredity, and Survival 'The cell is always speaking—the secret is to learn its language.' Bajer (1928– ) cell biologist.
Every one of us began with the fusion of a sperm and egg cell. © DENNIS KUNKEL MICROSCOPY, INC Nearly all multicellular organisms reproduce sexually by the fusion of an egg and a sperm. Like almost every cell in your body, this new cell—a zygote—has a full contingent of 23 pairs of chromosomes. But what about its parent cells, the sperm and egg? If the egg and sperm each had 23 chromosome pairs, their union would result in a zygote with 46 pairs—double the usual number. Theoretically, this cell would then grow into a person with 46 pairs of chromosomes per cell (rather than the usual 23 pairs). Subsequent generations would have even more chromosomes per cell.
Given the length of human history, can you imagine how many chromosomes our cells would have by now? Clearly, this is not what actually happens. Even early cell biologists realized that there must be a way to cut in half the number of chromosomes in egg and sperm cells. To accomplish that task, nature devised a special kind of cell division called meiosis. In preparation for meiosis, the chromosomes are copied once, just as for mitosis, but instead of one cell division, there are two. The result is four daughter cells, each containing 23 individual chromosomes rather than 23 pairs.
Meiosis is divided into chronological phases just like mitosis, and although the phases have the same names, there are some differences between them, especially in the early stages. Also, since there are two cell divisions in meiosis, each phase is followed by a I or II, indicating to which division it belongs. If mitosis is a show, then chromosomes are the stars. The main plot line is the even distribution of stars into two groups by the time the curtain goes down. But the stars play an unusually passive role. A director called the mitotic moves them from here to there on the cellular stage.
The mitotic spindle—a football-shaped array of fibers made of microtubules and associated proteins—forms at the beginning of mitosis between opposite ends, or poles, of the cell. The chromosomes (blue) become attached to the spindle fibers (green) early in mitosis. The spindle is then able to move chromosomes through the various phases of mitosis. How spindle fibers move chromosomes has captivated scientists for decades, and yet the answer remains elusive.
Conly Rieder, a cell biologist at the Wadsworth Center in Albany, New York, is investigating this challenging question. Some scientists believe that motor proteins act like cellular buses, conveying chromosomes along the fibers. Others, including Rieder, favor the idea that microtubules shrink or grow at their ends to reel in or cast out chromosomes. Still other scientists believe that the answer will come from combining both views. The potential applications of this molecular detective work are significant. When the spindle makes mistakes, chromosomes can end up in the wrong place, which may lead to cells with abnormal numbers of chromosomes. This, in turn, can cause serious problems, such as, cancer, or miscarriage, which, in 35 percent of cases is associated with cells carrying an atypical amount of genetic material.
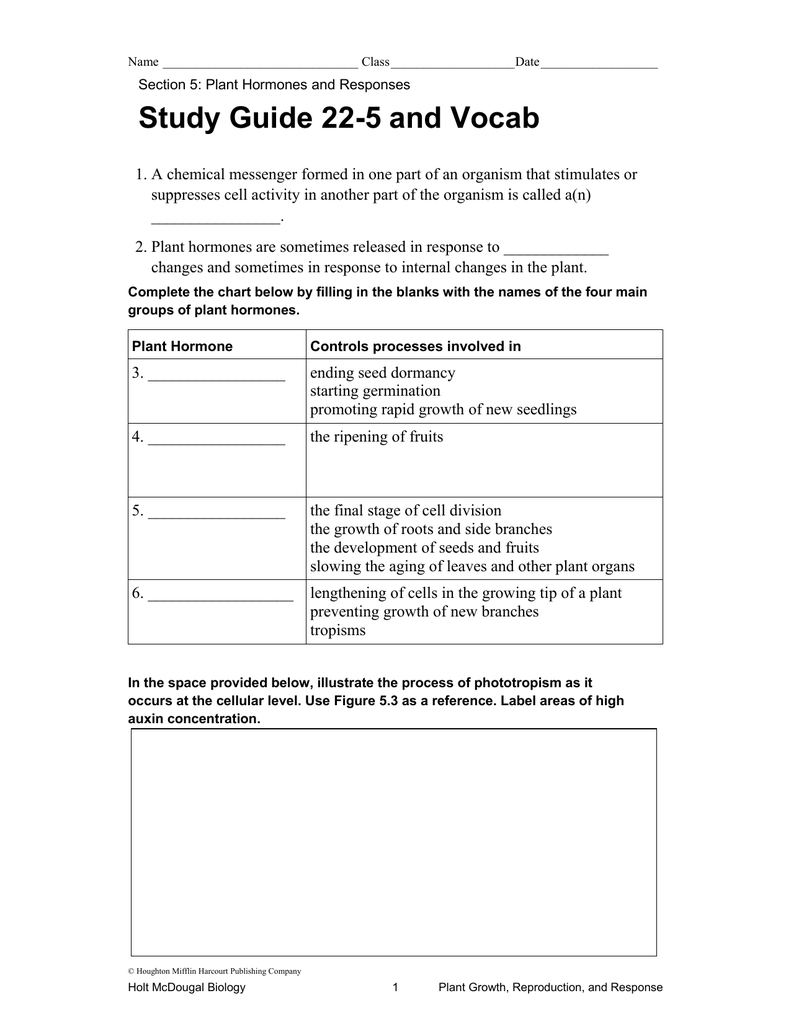
Phases of Meiosis. Men produce sperm continuously from puberty onward, and the formation of a sperm takes about a week.
The situation is quite different in women. Baby girls are born with a certain number of 'pre-egg' cells that are arrested at an early stage of meiosis. In fact, the pre-egg cell does not complete meiosis until after fertilization has occurred. Fertilization itself triggers the culmination of the process. This means that meiosis in women typically takes decades and can take as long as 40 to 50 years! Scientists have long suspected that this extended meiosis in women is responsible for certain genetic disorders in their children. The pre-egg cells have years in which to accumulate damaging mutations that may cause errors in the remaining steps of meiosis.
For example, the risk of Down syndrome, a common cause of mental retardation, increases in the babies of older mothers. The syndrome occurs when the chromosome 21 pair fails to separate during meiosis and both copies of the chromosome end up in a single egg cell. Subsequent fertilization by a sperm means that the resulting cell has three copies of chromosome 21 rather than the standard two.
No one knows exactly how or why the chromosomes fail to separate, and the question has been difficult to answer because of the lack of a suitable animal model in which to study the disorder. Now, Sharon Bickel, a molecular biologist at Dartmouth College in Hanover, New Hampshire, has developed a method that uses fruit flies to gain insight into this human puzzle. Fruit flies normally produce eggs continuously, but Bickel manipulated their diet in such a way as to suspend egg maturation, allowing the eggs to age.
This mimicked the aging of human eggs. Studying the aged fruit fly eggs, Bickel found that the incidence of problems in chromosome separation increased, just as it does in older women. Her work also indicated that a backup genetic system that helps to ensure proper chromosome separation and distribution deteriorates as fruit fly eggs age. No one yet knows if the same backup system exists in humans or if the same mistakes seen in the flies account for the increased risk of Down syndrome in the babies of older mothers. But the fruit fly model system will allow Bickel and others to investigate these important questions. Comparison Between Mitosis and Meiosis.
Chromosome Dancers Even members of the same family, who share much of their genetic material, can be dramatically different from one another. If you've ever been to a family reunion, you've seen living proof of this. How can the incredible diversity that we see in our own families, let alone in the world at large, be explained? Imagine 23 couples participating in a dance. The couples begin by lining up facing each other, forming two parallel lines.
Cell Growth And Reproduction Study Guide
It doesn't matter which line the dancers stand in, as long as they're across from their partners. Because men and women can be in either line, the dancers can line up in millions of different ways.
In fact, the number of possible arrangements is 2 23, or more than 8 million! You can think of the partitioning of the 23 pairs of chromosomes between the two daughter cells during the first cell division in exactly the same way: Each daughter cell will get one chromosome from each pair, but which one it gets is completely random. As we saw with the dancers, this generates over 8 million different combinations. This means that a single set of parents can produce over 64 trillion different zygotes! Meiosis can generate still more genetic variation through crossing over, during which chromosome partners physically swap sections with one another, generating hybrid chromosomes that are a patchwork of the original pair.
This rearrangement of the genetic material expands the number of possible genetic configurations for the daughter cells, further increasing diversity. You share some genes, and hence some physical traits, with your parents and your other relatives. But thanks to meiosis, you are a unique individual. So, thanks to the random splitting up of chromosome pairs and the genetic swapping that goes on during meiosis, you inherit a rather mixed bag of genes from your parents. This explains why family members can be so different from one another despite having a number of genes in common.
The genetic diversity brought to us courtesy of meiosis (and occasional genetic mutations) enhances the survival of our species. Having a widely varied pool of genes in a population boosts the odds that in the face of disease outbreaks or harsh environmental conditions, at least some individuals will have the genetic stuff it takes to survive—and pass on their genes. So in more ways than one, you have meiosis (and your parents) to thank for being here at all!